Open Journal of Mathematical Sciences
ISSN: 2523-0212 (Online) 2616-4906 (Print)
DOI: 10.30538/oms2019.0067
A mathematical model showing the potential of vitamin c to boost the innate immune response
Anuraag Bukkuri
Department of Mathematics, University of Minnesota, Minneapolis, MN 55455, USA.
\(^{1}\)Corresponding Author: bukku001@umn.edu
Abstract
Keywords:
1. Introduction
The innate immune response is an older evolutionary defense strategy against foreign bodies, and is the primary form of immunity in plants, fungi, insects, and primitive multicellular organisms. In humans, it provides the first line of defense from an infection, in a nonspecific manner. These responses are rapid and independent of the antigen. This is often contrasted with adaptive immunity, a key feature of which is the development of antibodies for a particular pathogen, leading to a "memory-based" evolutionary response.
Here, we will focus on the effect Vitamin C has on the innate immune system. To do this, we will first provide a short biochemical summary on interactions and dynamics involved in the innate response.
When a virus enters a body, it sends chemical signals to phagocytes, cells that protect the body by ingesting and degrading foreign bodies. When the phagocytes are activated, they release a set of oxidizing agents that kill the virus. Phagocytes specifically have three main mechanisms of killing a virus: oxygen-dependent, oxygen-independent, and via interferon-gamma.
In the oxygen-dependent process, once a phagocyte ingests a bacterium, its oxygen consumption increases, producing a toxic reactive oxygen species (ROS). These molecules are anti-viral/anti-bacterial [1], but are also harmful for the phagocyte. ROS is then used to kill the virus via mechanisms that will not be covered here.
The mechanisms behind the oxygen-independent process are omitted here, but it's important to understand that, as the name suggests, this does not cause production of ROS, but is also not as effective in killing bacteria/viruses as the oxygen-dependent process [2].
The extracellular interferon-gamma stimulates macrophages to produce nitric oxide, which kills the foreign body due to its toxicity. In some diseases such as chronic granulomatous disease, the efficiency of phagocytes is impaired, and recurrent bacterial infections are common [3]. In this disease, there are problems with oxygen-dependent killing, so the body must rely on the latter two mechanisms. Finally, in other congenital abnormalities, such as Chédiak-Higashi syndrome, there is defective killing of ingested microbes, so the body must solely rely on interferon-gamma to fight bacterial/viral infections [4].
If the phagocyte fails to engulf its target virus, it will, in a process called frustrated phagocytosis, release these toxic agents into its environment, thus harming the surrounding host cells. This is the source of several medical issues such as renal failure, acute lung injury, and liver damage--conditions we revisit later in this paper [5, 6, 7].
Vitamin C plays a great role in these processes in several different ways. One way is that it protects the host cells against the oxidants released by phagocytes [8, 9, 10, 11, 12, 13]. It also increases ROS production, which can be both beneficial and harmful for the immune system [14, 15, 16]. Vitamin C has been shown to increase mobility and chemotaxis of phagocytes [17, 18], as well as increase the interferon-gamma production [19]. Finally, it has been shown to reduce virus replication rates (though the presence of a virus also degrades the Vitamin C faster) [20, 21, 22].
Since Vitamin C plays a crucial role in the immune response in a variety of ways, the author hypothesized that it could be effectively used to treat some of the aforementioned diseases.2. Model Creation
From the above biochemical framework, the following mathematical model was constructed:2.1. Equation for Vitamin C (1)
It is well known that, unlike other plants and animals, humans cannot synthesize Vitamin C and must ingest it from their diet. Thus, the \(c_0\) term represents this external input of Vitamin C (from diet or treatment). The second term represents the natural degradation rate of of the Vitamin C in the body. The last term captures the oxidation of Vitamin C to dehydroascrobic acid, an oxidation reaction that occurs when Vitamin C is used for ROS sequestration, something which is triggered by the presence of viruses. Note that the carrying capacity of the viruses has been taken into account by including the \(V/V_{max}\) ratio.2.2. Equation for Phagocytes (2)
The first term in this equation represents the growth rate of phagocytes, incorporating their carrying capacity. The following terms represent the death of the phagocytes, both by natural causes, \(d_p\), and by the virus killing the phagocytes. The \(e\) parameter captures this killing rate, while the \(fC/C_M\) term is used to show how Vitamin C may enhance this killing. Note the use of carrying capacities for the virus and Vitamin C as well.2.3. Equation for Host Cells (3)
As in equation (1), the first term here represents the growth rate of the host cells, including their carrying capacity. The next terms are the death rates of the host cells, including natural death, killing by phagocytes (including enhancement of killing by Vitamin C), and killing by viruses.2.4. Equation for Viruses (4)
The first term represents the growth rate of the virus, including carrying capacity and Vitamin C's effect of reducing viral replication rates. The second term includes the natural death of the virus, phagocyte killing via oxygen-independent, oxygen-dependent, and interferon-gamma mechanisms, with the latter two methods being enhanced by Vitamin C.3. Parameter Estimation
Table 1 represents the base values of the parameters used in creating simulations of the dynamics among Vitamin C, phagocytes, host cells, and viruses under various medical conditions.Table 1. Parameters used in creating simulations.
Parameter | Meanings | Source | Value |
---|---|---|---|
\(c_0\) | Input of Vitamin C | [23] | 50 |
\(d_c\) | Vitamin C Degradation Rate | [24] | 0.05 |
\(C_M\) | Vitamin C Carrying Capacity | [25] | 2000 |
a | Loss of Vitamin C due to ROS sequestration | [26] | 0.1 |
b | Growth Rate of Phagocytes | [27] | 1.5 |
\(P_M\) | Phagocyte Carrying Capacity | Estimated | 400 |
\(d_p\) | Natural Death Rate of Phagocytes | Estimated | 0.1 |
e | Virus Killing Rate of Phagocytes | [1] | 0.5 |
f | Virus Killing Rate of Phagocytes Boosted by Vitamin C | [16] | 0.1 |
g | Growth Rate of Host Cells | Estimated | 1.5 |
\(H_M\) | Host Cell Carrying Capacity | Estimated | 1000 |
\(d_h\) | Natural Death Rate of Host Cells | Estimated | 0.1 |
h | Probability of Frustrated Phagocytosis | Estimated | 0.01 |
i | Killing of Host Cells by Phagocytes | [27] | 0.6 |
j | Protection of Host Cells from Phagocytes due to Vitamin C | [8] | 0.3 |
k | Killing of Host Cells by Virus | [28] | 0.4 |
l | Growth Rate of Virus | Estimated | 2.5 |
m | Reduction of Viral Growth Rate by Vitamin C | [22] | 1.25 |
\(V_M\) | Virus Cell Carrying Capacity | Estimated | 300 |
\(d_v\) | Natural Death Rate of Virus | Estimated | 0.1 |
z | Killing Rate of Viruses by Phagocytes | [16] | 0.6 |
p | Phagocyte Killing of Viruses Boosted by Vitamin C | [18] | 0.3 |
q | Oxygen-independent Killing by Phagocytes | [29] | 0.2 |
r | Oxygen-dependent Killing by Phagocytes | [1] | 0.6 |
s | Oxygen-dependent Killing Boosted by Vitamin C | [14] | 0.2 |
u | Interferon-gamma Killing by Phagocytes | [30] | 0.2 |
v | Interferon-gamma Killing Boosted by Vitamin C | [19] | 0.2 |
4. Model Results
First, using the above default parameter values, we simulate a viral infection in the body in three cases: with a constant stimulus of Vitamin C dosage to prevent any degradation, a constant stimulus of Vitamin C but allowing for some natural degradation, and a single dosage, which dies out. The following initial values were used: C = 2000, P = 300, H = 1000, V = 500 for the single treatment. For the continuous infusions, the same values were used, but C was set to 58 (the accepted physiological level). The results can be seen below.4.1. Control: Extreme Treatment - c0 = 100
Figure 1. Dynamics under Viral Infection with Extreme Constant Infusion of Vitamin C.
4.2. Control: Moderate Treatment - c0 = 90
Figure 2. Dynamics under Viral Infection with Moderate Constant Infusion of Vitamin C.
4.3. Control: Single Treatment - c0 = 0
Figure 3. Dynamics under Viral Infection with Single Vitamin C Dose
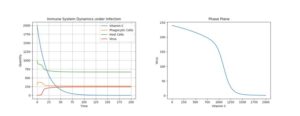
Figure 4. Dynamics with 1 Initial Virus Present with Single Vitamin C Dose
Figure 5. Phase Plane Comparison of 2000 Initial Vitamin C Dose (left) and 50 Initial Vitamin C Dose (right) under Viral Infection
5. Diseases
There are several diseases that are associated with imbalances in the innate immune system. In this section, the author will biologically explain what goes wrong in each situation, before mathematically proposing ways to treat them.6. Frustrated Phagocytosis
Phagocytes are part of the innate immune system and aim to engulf and absorb foreign particles such as bacteria, forming a phagosome. Normally, this phagosome moves toward the centrosome of the phagocyte, is fused with lysosomes, and forms a phagolysosome, leading to degradation. The phagolysosome is then acified, activating degradative enzymes [31, 32].
But if the phagocyte cannot engulf the foreign particle, it will release these particles into its environment--a process called frustrated phagocytosis. As would be expected, this process harms the surrounding host cells, causing many medical issues such as renal failure, acute lung injury, and liver damage [5, 6, 7]. To model these situations, we simply increase the value of h, the probability of frustrated phagocytosis, occurs to 0.7 (to clearly see the effects of extreme forms of these diseases). Note that the phase plane now compares host cells to Vitamin C concentrations. The two phase planes in Figure 6 show the effect of this disease on the host cell population.
Figure 6. Phase Plane Comparison of Healthy (left) and Frustrated Phagocytosis (right) States
Clearly, we can see that the host cell population in the second plot (the diseased state) is much lower than in the non-diseased state. It's this impact on the host cell tissue that causes diseases such as renal failure.
To see how to help this situation, let's consider what occurs in the presence of Vitamin C. Again, we'll consider the same 3 treatments as above. The same initial conditions as before were used (C set to 58 for continual infusions and to 2000 for single treatment).
6.1. Control: Extreme Treatment - c0 = 100
Figure 7. Dynamics under Frustrated Phagocytosis with Extreme Constant Infusion of Vitamin C
6.2. Control: Moderate Treatment - c0 = 90
Figure 8. Dynamics under Frustrated Phagocytosis with Moderate Constant Infusion of Vitamin C
6.3. Control: Single Treatment - c0 = 0
Figure 9. Dynamics under Frustrated Phagocytosis with Single Vitamin C Dose
Finally, consider the case, seen in Figure 9, of no treatment. In this case, the host cell concentration is greatly reduced down to under 500 cells, causing much damage to the underlying tissue. Also, the virus population is at a higher equilibrium at \(\approx 250\).
Thus, we have seen that, in cases like renal failure, acute lung injury, or liver damage, Vitamin C has the potential to limit tissue damage to the host cells greatly, a conclusion which is accord with current medical literature [33,34, 35, 36].
7. Chronic Granulomatous Disease (CGD)
In chronic granulomatous disease, the body has trouble producing the ROS that kill the pathogens. Specifically, this is due to defects in one of the four essential subunits of phagocyte NADPH oxidase. Due to this, the oxygen-dependent mechanism of phagocytic killing is essentially removed [37]. To model this disease, we simply set r and s (ROS killing of pathogens) to 0 and let f be 0 as well (since the ROS will not kill the phagocytic cells either). Then, we implement the 3 treatments as before. Here are the results:7.1. Control: Extreme Treatment - c0 = 100
Figure 10. Dynamics under CGD with Extreme Constant Infusion of Vitamin C
7.2. Control: Moderate Treatment - c0 = 90
Figure 11. Dynamics under CGD with Moderate Constant Infusion of Vitamin C
7.3. Control: Single Treatment - c0 = 0
Figure 12. Dynamics under CGD with Single Vitamin C Dose
8. Chédiak-Higashi Syndrome
In the Chédiak-Higashi syndrome, there exist mutations in the CHS1 gene, which provides instructions for making lysosomal trafficking regulators. These proteins play a role in transporting materials into the lysosome, which then break down toxic substances and digest the bacteria/viruses that enter the phagocytes [39].
Thus, in this disease, though the phagocytes can digest the bacteria, they cannot actually kill it. Due to this, we will multiply all the phagocyte-killing-bacteria terms by 0.1 to reflect this change. Then, for this case, we only run the extreme (left graph) and single treatment (right case) cases.
Figure 13. Dynamics under Chédiak-Higashi syndrome under Extreme Constant Infusion and Single Dose Vitamin C Treatments
9. Conclusion
In this paper, a mathematical model analyzing dynamics among Vitamin C, viruses, host cells, and phagocytic cells has been considered. Situations of viral infection, frustrated phagocytosis, chronic granulomatous disease, and the Chédiak-Higashi syndrome were considered, and the potential for Vitamin C to treat these conditions was considered. The model was examined under various conditions and was shown to be consistent with medical findings; effectiveness of Vitamin C as a treatment varied among diseases. The author hopes that this work will inspire future medical research that investigates the potential for the expansion of therapeutic use of Vitamin C to boost the immune system.Acknowledgments
The authors would like to express their thanks to the referee for his useful remarks.Author Contributions
All authors contributed equally to the writing of this paper. All authors read and approved the final manuscript.Competing Interests
The author(s) do not have any competing interests in the manuscript.References
- Dahlgren, C., & Karlsson, A. (1999). Respiratory burst in human neutrophils. Journal of Immunological Methods, 232, (1-2), 3-14. [Google Scholor]
- Ganz, T. (1999). Oxygen-independent microbicidal mechanisms of phagocytes. Proceedings of the Association of American Physicians, 111, (5), 390-395. [Google Scholor]
- Lipu, H.N., Ahmed, T.A., Ali, S., Ahmed, D., Waqar, M.A., Ahmed, A., & Ahmed, W. (2008). Chronic granulomatous disease. Journal Of Pakistan Medical Association, 58, (9), 516-518. [Google Scholor]
- Kaplan, J., De Domenico, I., & Ward, D.M. (2008). Chediak-Higashi syndrome. Current Opinion in Hematology, 15, (1), 22-29. [Google Scholor]
- Akaike, T., Suga, M., & Maeda, H. (1998). Free radicals in viral pathogenesis. Proceedings of the Society for Experimental Biology and Medicine,217, 64-67.[Google Scholor]
- Goode, H.F., & Webster, N.R. (1993). Free radicals and antioxidants in sepsis. Critical Care Medicine, 21,1770-1776. [Google Scholor]
- Peterhans, E. (1997). Oxidants and antioxidants in viral diseases. The Journal of Nutrition, 127, 962-965.[Google Scholor]
- Frei, B., England, L., & Ames, B.N. (1989). Ascorbate is an outstanding antioxidant in human blood plasma. Proceedings of the National Academy of Sciences of the United States of America, 86, 6377-6381. [Google Scholor]
- Hemilä, H. (1984). Nutritional need versus optimal intake. Medical Hypotheses, 14, 135-139.[Google Scholor]
- Hemilä, H. (1990). Auttaako C-vitamiini vilustumiseen? Duodecim, 106, 1306-1311. [Google Scholor]
- Hemilä, H. (1992a). Vitamin C and the common cold. British Journal of Nutrition, 67, 3-16.[Google Scholor]
- Hemilä, H. (1992b). Vitamin C, neutrophils and the symptoms of the common cold [letter]. The Pediatric Infectious Disease Journal , 11, 779 [Google Scholor]
- Hemilä, H. (2017). Vitamin C and Infections. Nutrients, 9, (4), 339. [Google Scholor]
- Corberand, J., Nguyen, F., Fraysse, B., & Enjalbert, L. (1982). Malignant external otitis and polymorphonuclear leukocyte migration impairment. Improvement with ascorbic acid. Archives of otolaryngology, 108, 122-124. [Google Scholor]
- Ganguly, R., Durieux, M.F., & Waldman, R.H. (1976). Macrophage function in vitamin C-deficient guinea pigs. The American Journal of Clinical Nutrition, 29, 762-765. [Google Scholor]
- Levy, R., & Schlaeffer, F. (1993). Successful treatment of a patient with recurrent furunculosis by vitamin C: Improvement of clinical course and of impaired neutrophil functions. International Journal of Dermatology, 32, 832-834.[Google Scholor]
- Goetzl E.J., Wasserman, S.I., Gigli, I., et al. (1974). Enhancement of random migration and chemotactic response of human leukocytes by ascorbic acid. The Journal of Clinical Investigation, 53, 813-818. [Google Scholor]
- Goldschmidt, M. C.(1991). Reduced bactericidal activity in neutrophils from scorbutic animals and the effect of ascorbic acid on these target bacteria in vivo and in vitro. The American Journal of Clinical Nutrition, 54, 1214-1220. [Google Scholor]
- Dahl, H., & Degre, M. (1976). The effect of ascorbic acid on production of human interferon and the antiviral activity in vitro. Acta pathologica et microbiologica Scandinavica,1976, (84b), 280-284. [Google Scholor]
- Atherton, J.G., Kratzing, C.C., & Fisher, A. (1978). The effect of ascorbic acid on infection of chick-embryo ciliated tracheal organ cultures by coronavirus. Archives of Virology, 56, 195-199 [Google Scholor]
- Bissell, M.J., Hatie, C., Farson, D.A., et al. (1980). Ascorbic acid inhibits replication and infectivity of avian RNA tumor virus. Proceedings of the National Academy of Sciences of the United States of America, 77, 2711-2715. [Google Scholor]
- Harakeh, S., Jariwalla, R.J., & Pauling, L. (1990). Suppression of human immunodeficiency virus replication by ascorbate in chronically and acutely infected cells. Proceedings of the National Academy of Sciences of the United States of America, 87, 7245-7249. [Google Scholor]
- German Nutrition Society (DGE) (2015). New Reference Values for Vitamin C Intake. Annals of Nutrition and Metabolism, 67, 13-20. [Google Scholor]
- Matei, N., Birghila, S., Popescu, V., Dobrinas, S., Soceanu, A., Oprea, C., & Magearu, V. (2008). Kinetic study of vitamin C degradation from pharmaceutical products. Romanian Journal of Physics, 53, (1-2), 343-351. [Google Scholor]
- Padayatty S.J., & Levine, M. (2016). Vitamin C: the known and the unknown and Goldilocks. Oral Diseases, 22, (6), 463-469. [Google Scholor]
- Zhong, X., Zeng, M., Bian, H., Zhong, C., & Xiao, F. (2017). An evaluation of the protective role of vitamin C in reactive oxygen speciesinduced hepatotoxicity due to hexavalent chromium in vitro and in vivo. Toxicology, 12, (15), 1-12. [Google Scholor]
- Lin, J.J., Grinstein, S., & Roth, Z. (2017). Diversity and Versatility of Phagocytosis: Roles in Innate Immunity, Tissue Remodeling, and Homeostasis. Frontiers in Cellular and Infection Microbiology, 7, 191.[Google Scholor]
- Roulston, A., Marcellus, R.C., & Branton, P.E. (1999). Viruses and apoptosis. Annual Review of Microbiology, 53, 577-628. [Google Scholor]
- Hoffbrand A.V., Pettit, J.E., & Moss, P.A. (2005). Essential Haematology (4th ed.). London: Blackwell Science. [Google Scholor]
- Marchi, L.F., & Mantovani, B. (2014). Interferon-gamma enhances phagocytosis, the production of reactive oxygen species and pro-inflammatory cytokines--implications for innate and acquired immunity. Inflammation and Cell Signaling, 1, 113. [Google Scholor]
- Gordon, S. (2016). Phagocytosis: An Immunobiologic Process. Immunity, 44, (3), 463-475. [Google Scholor]
- Flannagan, R.S., Jaumouille, V., & Grinstein, S. (2012). The Cell Biology of Phagocytosis. Annual Review of Pathology: Mechanisms of Disease, 7, 61-98. [Google Scholor]
- Marin, J.J.G., Perez, M.J., Serrano, M.A., & Macias, R.I.R. (2018). Chapter 13 - Chemoprotective Role of Vitamin C in Liver Diseases. The Liver: Oxidative Stress and Dietary Antioxidants, 139-153. [Google Scholor]
- Bharara, A., Grossman, C., Grinnan, D., Syed, A., Fisher, B., DeWilde, C., Natarajan, R., & Fowler, A.A.B. (2016). Intravenous Vitamin C Administered as Adjunctive Therapy for Recurrent Acute Respiratory Distress Syndrome. Case Reports in Critical Care, 1-4. [Google Scholor]
- Deicher, R., & Horl, W.H. (2003). Vitamin C in chronic kidney disease and hemodialysis patients. Kidney and Blood Pressure Research, 26, (2), 100-106.[Google Scholor]
- McHugh, G.J., Graber, M.L., & Freebairn, R.C. (2008). Fatal vitamin C-associated acute renal failure. Anaesthesia and Intensive Care, 36, (4), 585-588. [Google Scholor]
- Deffert, C., Cachat, J., & Krause K.H. Phagocyte NADPH oxidase, chronic granulomatous disease and mycobacterial infections Cellular Microbiology, 16, (8), 1168-1178.[Google Scholor]
- Anderson, R. (1981). Assessment of oral ascorbate in three children with chronic granulomatous disease and defective neutrophil motility over a 2-year period. Clinical & Experimental Immunology, 43, 180-188. [Google Scholor]
- Al-Tamemi, S., Al-Zadjali, S., Al-Ghafri, F., & Dennison, D. (2014). Chediak-Higashi syndrome: novel mutation of the CHS1/LYST gene in 3 Omani patients. Journal of Pediatric Hematology/Oncology, 36, (4), 248-250.[Google Scholor]
- Hughes, D.A. (1999). Effects of dietary antioxidants on the immune function of middle-aged adults. Proceedings of the Nutrition Society, 58, (1), 79-84.[Google Scholor]
- Carr, A.C., & Maggini, S. (2016). Vitamin C and Immune Function. Nutrients, 8, 1-24. [Google Scholor]
- (1977). Ascorbate and Chediak-Higashi Syndrome. Nutrition Reviews, 35 (7), 170-172. [Google Scholor]
- Gallin, J. I., Elin, R.J., Hubert, R.T., Fauci, A.S., Kaliner, M.A., & Wolff, S.M. (1979). Efficacy of Ascorbic Acid in Chediak-Higashi Syndrome (CHS): Studies in Humans and Mice. Blood, 53, (2), 226-234. [Google Scholor]